We all rely on carbon steel (CS). Whether in commuter structures, pipelines, wind turbines, oil rigs, or buildings. CS must be strong and secure, therefore the need to thoroughly and effectively inspect carbon steel structures for defects is essential.
Why? CS is usually easy to weld, doesn’t cost too much, and it’s extremely reliable. Carbon steel can be segmented into four main categories: low carbon steel (sometimes known as mild steel), medium carbon steel, high carbon steel, and ultra-high carbon steel.
Low Carbon Steel
Typically, low CS has a carbon range of 0.05–0.25%. This is one of the largest groups of CS. It can take many shapes: from flat sheets to structural beams. Depending on the desired properties of mild steel, other elements can be added or increased in the steel. For example, in drawing-quality low CS, the carbon level is kept low and aluminum is added. This grade of steel is more ductile than commercial-quality CS and it is suited to producing deep-drawn parts or other parts needing severe deformation.
In the case of structural steel, the carbon level is higher and the manganese content increased to improve the hardening depth, strength, and toughness of the steel. This grade of CS is used to make structural steel shapes such as I-beams.
Medium Carbon Steel
Typically, medium CS has a carbon range of 0.30–0.60% and a manganese content range of 0.06–1.65%. This grade is stronger than low carbon steel, but it is more difficult to form, weld, and cut than mild steel. Medium CS is quite often hardened and tempered using heat treatment. This grade balances ductility and strength, and offers a good resistance to wear. Medium CS is used for large parts, forging, and automotive components, among others.
High Carbon Steel
Commonly known as carbon tool steel, this grade typically has a carbon range of 0.70–2.50%. High CS is very difficult to cut, bend, and weld, and once heat treated, it becomes extremely hard and brittle. Very strong, it is used for springs, swords, and high-strength wires.
Ultra-High Carbon Steel
This grade of CS has a carbon range of approximately 2.50–3.00%. This grade can be tempered to great hardness and is used for special purposes like non-industrial knives, axles, and punches.
Of course, carbon steel is not impervious to defects. They are usually the result of one or several of the following processes:
Segregation
In metallurgy, this is a process where one component of an alloy or solid solution separates in small regions within the solid or on the solid’s surface. Segregation causes irregularities in the properties of the carbon steel, usually adverse to the quality of the metal, and zones of reduced corrosion resistance in the immediate vicinity of segregation.
Porosity
This is defined as void spaces on or in the material. It appears often in castings. In weld metal, it’s usually the result of gas being trapped inside. In castings, Often, porosity is the root cause of coating failures, which manifest themselves as surface pitting, spotting, and corrosion.
Shrinkage (or Hot Cracking)
This is the formation of cracks during the solidification of metal. The process is also known as hot shortness, hot fissuring, solidification cracking, and liquation cracking. Hot cracking usually occurs when the available supply of liquid weld metal is insufficient to fill the space available to solidifying metal.
Inclusions
These are particles, larger than ~0.5 µm, generally found in all steels, even the very purest. The most common inclusions in steel are oxides and sulphides, but also include nitrides and silicates, which are all non-metallic. Such inclusions can lead to cause cracks such as sulphide-stress cracking (SSC) and fatigue failures.
Residual Stresses
Residual stresses are the stresses remaining in a solid material after their original cause is removed. These stresses, if uncontrolled, can lead to the premature failure of critical components.
Embrittlement
This process is a loss of material ductility, making it brittle and prone to cracking. The absorption of hydrogen sulfide, leading to SSC, embrittles carbon steel. Similarly, CS absorbing hydrogen leads to hydrogen embrittlement, which can cause the metal to fracture.
Progressive and localized structural damage occurring when a material is subjected to cyclic loading. If the loads are above a certain threshold, microscopic cracks begin to appear at stress concentrators such as the surface, persistent slip bands, and grain interfaces. Eventually, cracks reach a critical size, propagating suddenly, resulting in failure.
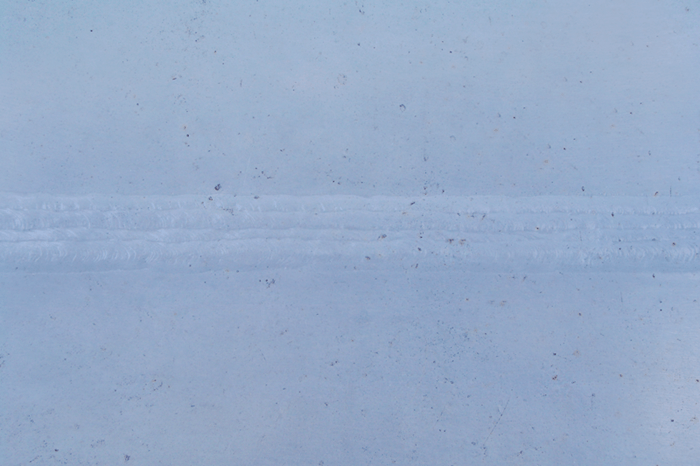
Cracking
Cracking is the most prevalent type of defects in in-service carbon steel welds. Cracks come in various shapes and sizes, from surface-breaking cracks to stress-corrosion cracking (SCC). Some of the challenges of inspecting CS surfaces for defects are that they are often rough (hard on surface probes) and very often coated with paint or other types of protective coatings. These often need to be removed to perform surface inspections and then reapplied, which is both costly and time consuming, not to mention harmful to the environment.
Cracking includes:
- Longitudinal cracks: Breaks in the surface parallel to the weld axis that may be along the centerline of the weld, close to the weld toes, or in the heat-affected zone (HAZ), for example.
- Transverse cracks: Breaks in the surface perpendicular to the weld axis that may be completely within the weld metal or may extend from the weld metal into the base metal.
- Crater cracks: Breaks in the surface that occur at the crater of a weld because welding was improperly terminated. Crater cracks are also referred to as star cracks.
- Branching cracks: Clusters of connected cracks originating from a common crack.
- Stress-corrosion cracking: Crack growth leading to the failure of metals under the combined action of corrosion and stress.
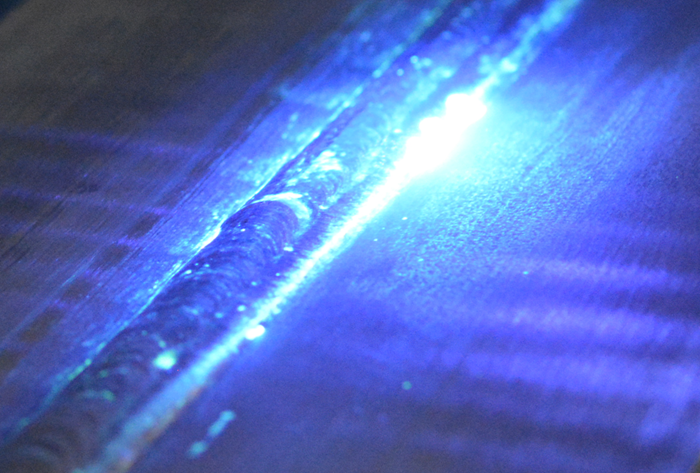
As mentioned above, a number of other types of defects can develop in base metal carbon steel and CS welds:
- Corrosion: Gradual material destruction by chemical reactions with the surrounding environment
- Surface porosity: Gas pores that break the carbon steel surface
- Lack of fusion: Poor adhesion of the weld bead to the base metal
- Pitting: Form of extremely localized corrosion leading to the creation of small “pits” in the metal
- Dents: Depressions in surfaces from pressure or blows
To prevent failures and prolong the life of assets, structures, and equipment, carbon steel must be regularly inspected for defects. There are several standards governing this activity, published by ASTM International, the American Society of Mechanical Engineers (ASME) and the International Standards Organization (ISO). These standards state that ultrasonic testing (UT), eddy current testing (ECT, including eddy current array), magnetic particle testing (MT), and liquid penetrant testing (PT) may be used to inspect for surface-breaking defects, according to specific guidelines.
ASTM E3052-16
ASTM E3052-16 covers the use of ECA sensors for the non-destructive testing of carbon steel welds. It includes the detection and sizing of surface-breaking cracks in joints, accommodating for non-magnetic and non-conductive coatings up to 5 mm (0.197 in) thick (typical) between the sensor and the joint. The practice covers a variety of cracking defects, such as fatigue cracks and other types of planar discontinuities, at various locations in the weld (heat-affected zone, toe area, and weld cap, for example). It covers the length and depth sizing of such surface-breaking discontinuities.
ASME’s Boiler and Pressure Vessel Code Case 2235-9
ASME’s Boiler and Pressure Vessel Code Case 2235-9 states that unless UT data analysis confirms defects are not surface connected, said defects are considered surface-breaking, which is unacceptable unless a surface inspection using MT, PT, or eddy current testing (ECT) is performed. The document also mention that all relevant eddy current testing indications open to the surface are unacceptable regardless of their length.
ISO BS EN ISO 5817:2007
Similarly, in ISO’s BS EN ISO 5817:2007, Welding — Fusion-welded joints in steel, nickel, titanium and their alloys (beam welding excluded) — Quality levels for imperfections, ECT methods are cited as good surface-breaking and near-surface defect inspection methods, mainly in ferritic materials (welds, heat-affected zones, parent materials). The document also sets the minimum size of defects ECT methods should be able to detect in ferritic steel welds (as welded) as 1 mm (0.039 in) deep and 5 mm (0.197 in) long.
As you might expect, these [inspection methods have their advantages and disadvantages](Similarly, in ISO’s BS EN ISO 5817:2007, Welding — Fusion-welded joints in steel, nickel, titanium and their alloys (beam welding excluded) — Quality levels for imperfections, ECT methods are cited as good surface-breaking and near-surface defect inspection methods, mainly in ferritic materials (welds, heat-affected zones, parent materials). The document also sets the minimum size of defects ECT methods should be able to detect in ferritic steel welds (as welded) as 1 mm (0.039 in) deep and 5 mm (0.197 in) long.
As you might expect, these inspection methods have their advantages and disadvantage, making one suited to some applications and others not, but they are all the best way to detect and monitor the progression of defects in assets and an essential part of asset integrity management (AIM).
Wrapping Up
Identifying the type of defect and understanding its root cause are essential in finding and monitoring defects in assets, which we explore in 4 Methods of Detecting Surface Defects in Carbon Steel. Don’t be afraid to leave your questions in the comments!