Skin Depth
Before we get into the thick of it, it’s important to understand the principle of skin depth (sometimes referred to as penetration depth). It’s greater at a low current frequency, conductivity, and permeability. As they increase, skin depth decreases. You don’t normally have control over conductivity and permeability. Therefore, the frequency of the alternating current running through the wire coil is your best ally. The type of defects you’re looking for and, more importantly, their location in the material guide you in selecting a frequency that will yield adequate penetration.
Check out my colleague Marc Grenier’s article on basics to learn more. What you need to take away from the article: eddy currents induced in ferromagnetic materials tend to stay near the surface and are unable to reach deeper defects.
Countering the Adverse Effects of Magnetism on Skin Depth
The good news is, despite what I’ve said, there are ways to counteract the negative effects of ferromagnetic materials on eddy current penetration.
To enable eddy currents to reach deeper down in the ferromagnetic material under test, you can saturate it with a magnetizing field. This makes the relative permeability drop and increases the skin depth, proportionally.
Consider the magnetization curve of duplex stainless steel in the figure below.
As you can see, the relative permeability of the material increases the more the magnetizing field increases until it peaks. Then, it begins to drop. The material is considered saturated when its relative permeability reaches 1. In practice, an acceptable level of magnetic saturation enables eddy currents to penetrate deep enough to detect the target defects.
The shape of the relative permeability curve of all mildly ferromagnetic materials is the same. The only differences are the location of the peak and the intensity of the magnetizing field necessary to reach it. Some materials, like MONEL®, require a relatively weak magnetizing field to lose their ferromagnetic properties. Others, like stainless steel grade 439, require a much stronger field.

Using Magnetic Saturation in Mildly Ferromagnetic Tube Inspection
In tubing inspections, magnetic saturation bobbin probes apply this principle. They incorporate strong magnets in their heads, where the bobbin coils are located. This enables the probe to generate the necessary magnetizing field to saturate tubes. For given grades, the larger magnets are, the stronger the magnetic field they produce. Unfortunately, magnetic saturation doesn’t only depend on the size of the magnet inside the tube. As I mentioned above, it also depends on the tube material and its wall thickness. The intensity of the magnetizing field decreases the further away from the magnet. This holds true for tubesheets and support plates, too, where the added material makes the probe blind to defects because they are too thick to saturate.
Of course, the size of magnets in any given probe is mostly limited by its diameter. That’s why smaller magnetic saturation probes produce weaker magnetic fields. It follows that in certain cases, specific combinations of magnet dimensions, tube material, and wall thickness don’t weaken permeability enough for eddy currents to adequately penetrate the material.
Sample Inspection in a Carbon Steel Tube
The following data was obtained with a 14.80 mm (0.583 in) saturation probe in a 19.05×2.11 mm (0.749×0.083 in) carbon steel tube. Carbon steel has a very high relative permeability. It’s therefore not typically inspected with magnetic saturation probes. The test tube had three outer diameter (OD) defects of different depths and one through-wall hole.
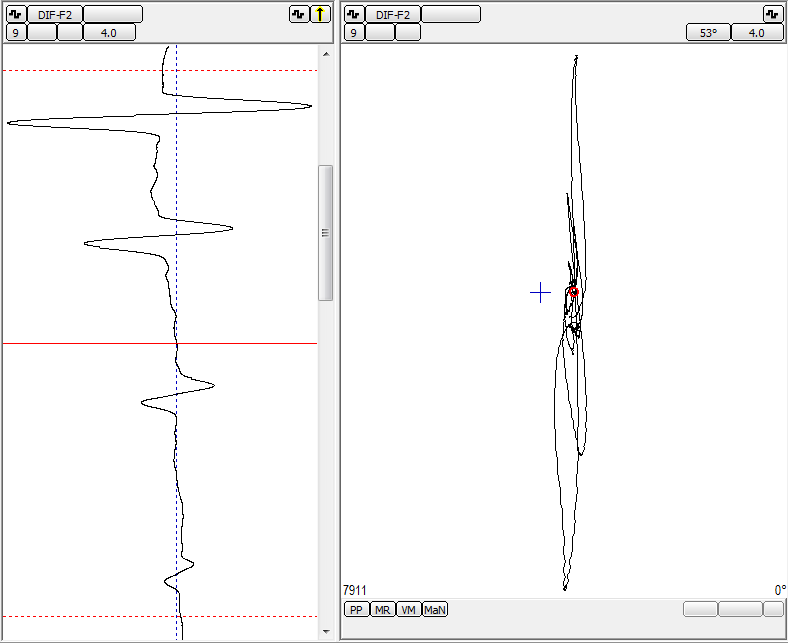
As you can see, the defects detected by the probe showcase almost no phase angle difference. This makes them hard to differentiate, but these are the kind of results to expect when the relative permeability of the material is high (because eddy currents remain too close to the surface).
Detection Anomaly
The fact that OD defects are detected may seem puzzling. How can they be when eddy currents are not supposed to reach them? Well, even though eddy currents remain near the surface, the loss of material concentrates the probe’s magnetic field into a smaller volume. This locally affects the relative permeability and the probe detects the variations indirectly. Although this is not what magnetic saturation probes are designed for, this concentration phenomenon opens the door to inspecting ferromagnetic tubes with them. It also means that the probes will only offer detection capabilities—they can’t be used for sizing and the inspection performance is a bit touch and go. It depends on the combination of probe diameter and tube properties. Tread with care.
Sample Inspection in a Duplex Stainless Steel Tube
Under appropriate conditions, the probe magnets are powerful enough for eddy currents to completely penetrate the thickness of tubes and yield sizing information. To illustrate, here is the differential channel of a 14.80 mm (0.583 in) saturation probe in a 19.05×2.11 mm (0.749×0.083 in) duplex stainless steel tube. Just like the carbon steel tube used above, this one includes three OD defects of different depths and one through-wall hole. You can see here that each defect displays a clear phase angle used to size the defects.
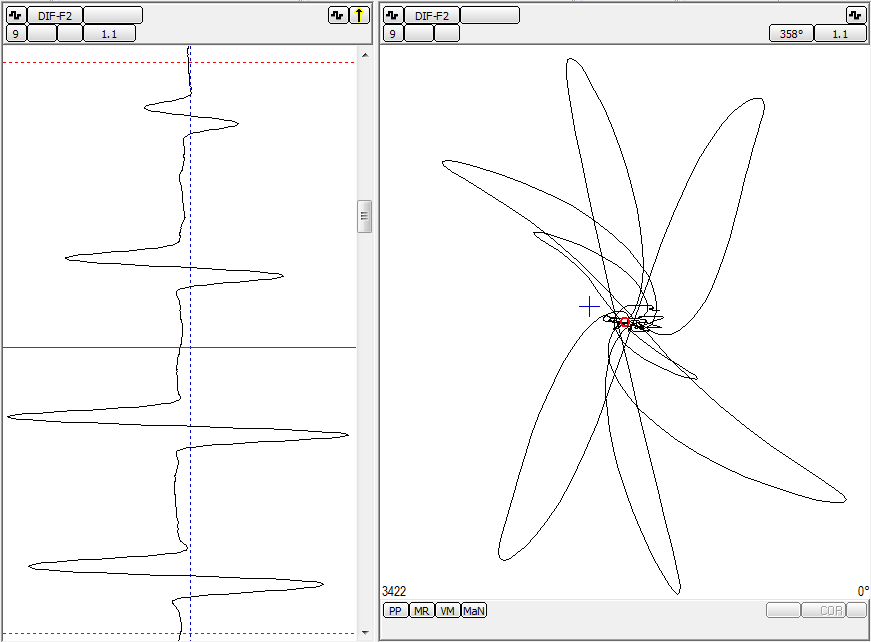
The Takeaway
Using magnetic saturation bobbin probes in ferromagnetic tubing has many advantages. They include the capacity to detect small pits and offer precise depth sizing. The probes are used exactly the same way as conventional ECT probes. They therefore require no special training for people already familiar with ECT.
There are, however, some situations where magnetic saturation probes won’t be optimal. The cause being the combination of the size of probe magnets, tube material, and wall thickness of the tube. Thick tubes with high relative permeability are difficult to saturate, more so when they have small inner diameters.
Selecting the Right Probe
The following table illustrates different combinations of tube properties. It suggests what magnetic saturation probes you should be using in specific situations. It was elaborated through computer simulations—actual results may differ. It’s still a reference to make sure whether the probe you’ve chosen is near the limit where it won’t be able to completely saturate your tubes. Yellow combinations represent borderline cases where adequate saturation is theoretically almost achieved. Even though the materials in the table are all mildly ferromagnetic, magnetic saturation probes are very efficient at removing the noise from internal ferromagnetic deposits.
As you might have expected, stainless steel grade 439 (which has a high relative permeability) is harder to saturate than MONEL (which has a relative permeability close to 1). You can also see that saturation limits are where smaller probes are used in thicker tubes.
Other inspection techniques such as high-frequency remote-field testing (RFT) are viable alternatives to magnetic saturation in ferromagnetic tubes. That’s because they can detect and size ID and OD defects with accuracy. Technologies like near-field testing (NFT) and near-field array (NFA) are also viable choices, if you are mainly preoccupied with detecting defects.
